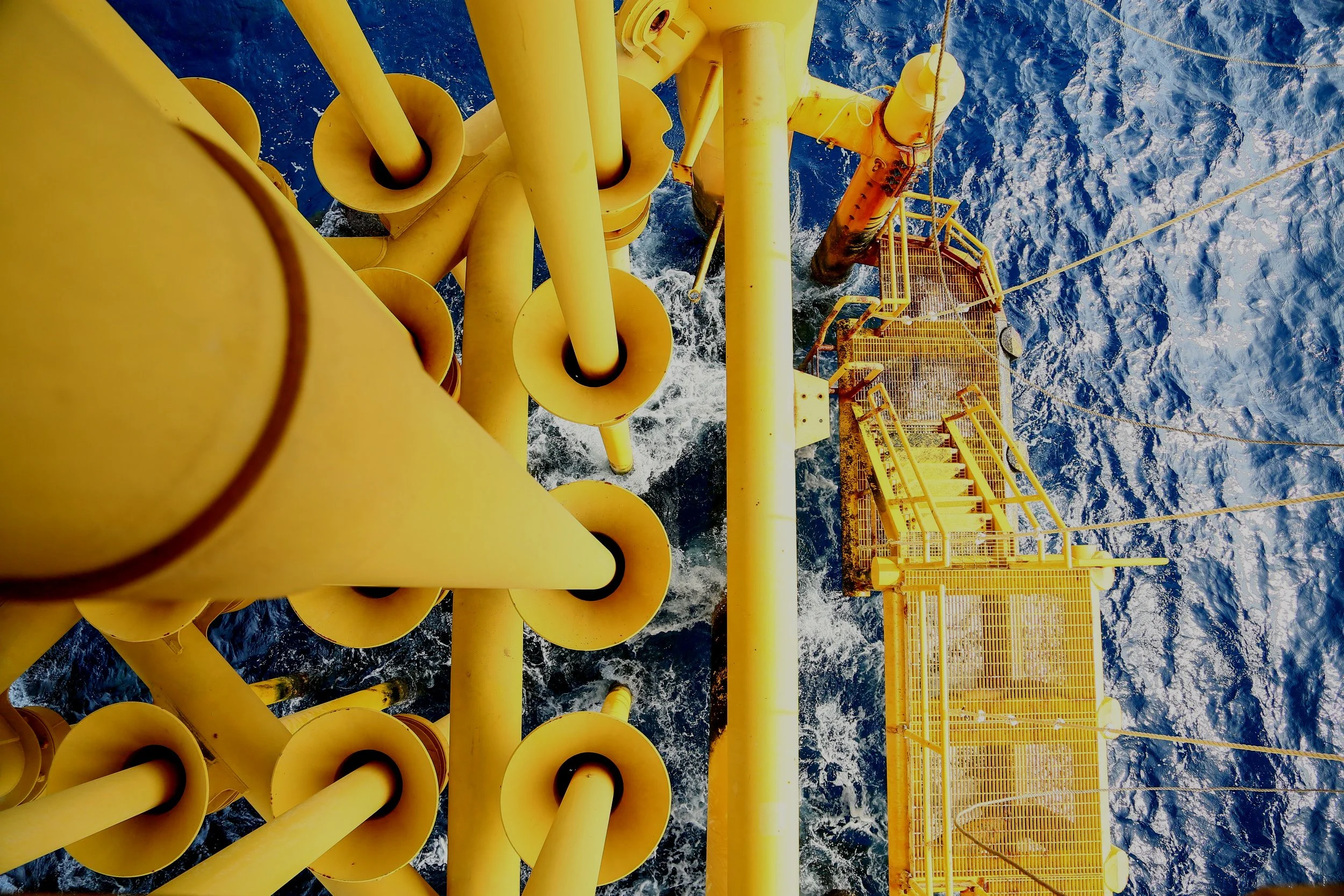
System Integration in the North Sea
Offshore system integration includes strategically coupling offshore sectors by integrating infrastructures, services, and logistics and multifunctional use of space (spatial integration). Technologies and concepts that are topics of detailed studies in the North Sea Energy program are:
Offshore system integration includes strategically coupling offshore sectors by integrating infrastructures, services, and logistics and multifunctional use of space (spatial integration). Technologies and concepts that are topics of detailed studies in the North Sea Energy program are:Offshore power-to-hydrogen on (existing gas) platforms and energy islands with transport with the use of (existing hydrocarbon) pipelines;
Offshore power-to-methanol and -ammonia on (existing gas) platforms and energy islands;
Energy storage; either in the form of greenfield applications or by using existing offshore legacy hydrocarbon assets (platforms, pipelines, wells, and reservoirs).
Offshore energy hubs: spatial and technical integration of various offshore commodities, also allowing for service and logistics sharing.
Electrification of (new and existing) platforms to decrease offshore gas consumption and CO₂ (and other) emissions and to feed other future activities (power-to-hydrogen, CCS) with clean energy;
Carbon transport and storage using offshore power infrastructure, (existing gas) pipelines and/or ships, and depleted hydrocarbon fields;
Service and logistics sharing between sectors to reduce costs, environmental pressure (emissions and disturbance), and pressure on human capital.
These technologies and concepts are described in more detail below.
Offshore System Integration Concepts
Power-to-hydrogen
Power-to-hydrogen concerns the conversion of electricity to hydrogen gas. Purified (sea)water is split into hydrogen and oxygen with the input of electricity. Power-to-hydrogen is interesting to allow sectors that cannot (fully) be electrified to decarbonise and as one of the attractive methods to offer flexibility to the energy system. It offers added value because the timing of energy production and use can be decoupled. Furthermore, future large-scale storage of hydrogen is possible at competitive costs compared to other means of energy storage. Hydrogen storage is foreseen at very large scales in subsurface caverns or depleted gas fields (ca 0.1–10 TWh). This could offer security of demand and supply in an energy system with a high supply of intermittent sustainable electricity.
Offshore the main source of electricity is foreseen to be offshore wind, but future production could in also include offshore solar or other power sources. The main offshore locations for conversion are considered to be existing and new platforms and/or energy islands. However, a very important limiting factor is the space available on platforms to reach economies of scale. Other important risks also need to be managed: technical risks, economical risks, commercial risks, organizational risks, and regulatory risks. Hydrogen production onshore is currently demonstrated in units with typical sizes of 1-10 MW. The application offshore is currently very novel and the first pilots at MW scale are being launched in the next year(s).
The main argument for moving hydrogen production offshore is to save electrical infrastructure costs and reduce conversion and transport losses of electricity. Transport of hydrogen through (existing) pipelines offshore has lower costs in comparison with offshore electricity transmission. However, this requires that the costs of offshore hydrogen are not disproportionately high in comparison with onshore hydrogen production. Further arguments are that offshore hydrogen production could benefit from already available gas infrastructure that further lowers the cost of transport; that the transport capacity of gas infrastructure benefits from economies of scale; and that it has better flexibility. Permitting lead times can be shorter, especially for existing pipelines, and onshore grid congestion can be alleviated. The spatial pressure of realising and landing electricity cables to shore could be alleviated. Placing conversion and storage offshore may ease implementation in comparison with onshore projects in relation to spatial planning and public perspectives; especially when scaling up electrolysis capacity to the scale of hundreds of MW or GW. Offshore hydrogen production may also reduce curtailment of offshore electricity production and thus make more efficient use of offshore installed wind (and solar) capacity and the space they claim.
Worlds first offshore hydrogen production pilot
The first North Sea Energy spin-off project PosHYdon will pilot offshore green hydrogen production at 1 MW scale at the operational Q13a-A platform. This is an already electrified platform located near the Dutch coast, 13 kilometres from Scheveningen. Production is expected to start in 2023.
Q13a-A platform that will host the PosHydon 1 MW Offshore Electrolyser Project © Neptune
Power-to-x
Besides power-to-hydrogen, renewable electricity can also be converted into other gases or liquids with existing markets and market value. In this phase of the program, we investigated the use of hydrogen in the production process of ammonia and methanol. Methanol requires CO₂ in the production process, which could be sourced from CO₂ infrastructure. Ammonia synthesis requires nitrogen production from ambient air. The production of power-to-x at an offshore location requires a substructure, either platform or island, that will be able to offer a multitude of functionalities. Transport is then possible via pipelines or ships.
Energy Storage
One of the possible applications to offer flexibility to the energy system is storage. This may be done on a small(er) scale in electricity storage technologies on existing platforms (batteries), at the seabed or shallow subsurface (e.g. compressed air, hydro), or in the form of gas storage (hydrogen) in small tanks, caverns or gas fields. This could be placed on, or unlocked via, platforms or islands. Both electricity storage options and integrated power-to-hydrogen solutions have specific advantages and disadvantages for providing flexibility to the electrical system. The biggest advantage of power-to-hydrogen is its potential integration into the gas system and access to the large flexibility of that system, whereas electricity storage technologies are suitable for fast response and high cycling rates and generally have higher roundtrip efficiencies.
Visit the map on offshore energy storage potential in the North Sea Energy atlas
Offshore energy hubs
North Sea Energy Hubs can be important stepping-stones for large-scale system integration and therefore are one of the central elements in the North Sea Energy program. Energy hubs are defined as offshore energy systems where the production, conversion, and/or storage of energy commodities (electricity, natural gas, hydrogen) and CO₂ are co-located. Transport of energy commodities and CO₂ to and from shore takes place via national transport corridors and/or via international interconnections. In this way, energy hubs are search areas for offshore system integration opportunities, i.e., where activities such as electricity production, CO₂ transport and storage, offshore hydrogen production, and platform electrification for (green-field) natural gas production can be combined. This can be developed on one or a combination of different structures offshore, for example, platforms, energy islands, or other types of installations.
For platform-based hubs, a 500 MW design of offshore hydrogen production was developed as part of this phase of the program. Offshore energy islands are currently considered as an option to enable cost-effective onshoring of large amounts of offshore wind energy. An important energy system service function considered for the islands is the conversion of alternating current (AC) to direct current (DC), which allows for the transport of vast amounts of electricity to shore over longer distances. Interconnection and hydrogen production are also identified as interesting use functions of such offshore islands that could improve the economic viability of the hub. Typically, multiple foundation and construction types of energy hubs can be discerned, including sand-, caisson- and platform-based structures or hybrids. Within this phase of the North Sea Energy program, the sand-based and hybrid structure was studied in more detail.
Visualisation of the North Sea Energy 500 MW Hydrogen Production Platform and Concept for an Offshore Energy Island (Sand Based)
Platform electrification
Electrification of hydrocarbon production platforms concerns electrification of the energy demand of these platforms (mainly processing and compression energy). Currently, this energy is primarily being supplied by gas-fired turbines. Electrification replaces the relatively inefficient gas turbines or engines installed on the platform and thereby reduces overall energy consumption and CO₂ and NOx emissions. Furthermore, electrified production platforms could be important stepping-stones for other system integration concepts, such as power-to-hydrogen and carbon capture and storage. A cabled connection to an offshore power hub provides a reliable, stable power supply and improves the security of supply. Decentral electrification of platforms with wind, solar, wave, and tidal energy is also being studied and developed on the North Sea.
Platform electrification – three examples in the Dutch North Sea
While platform electrification is common in Norway, in other countries of the North Sea this is still rather novel. In the near future, three platforms are set to be electrified in the Netherlands. The Q13 platform and Ameland Westgat platform are already electrified with a land connection (the latter is planned to be ready in 2022). A greenfield offshore gas production project that will be electrified is the N05-A platform which will operate from the end of 2024 on wind energy from the nearby (German) Riffgat wind farm.
Visualisation of the N05-A Platform and the Connection to the Riffgat Wind Park © One-Dyas
Carbon capture and storage
CCS typically involves CO₂ capture, compression of CO₂ from gas to liquid phase or a denser gas, and transport and isolation from the atmosphere by storage. Transport of CO₂ between sources and storage sites will occur most likely by pipeline or by ship. Storage takes place by injecting CO₂ into porous rocks in, for example, (near) empty gas fields or deep aquifers (saline formations). CCS requires a transport and storage infrastructure to be developed in the North Sea. An important aspect is the possibility to use parts of the existing oil and gas infrastructure (wells, platforms, pipelines) for CCS, which become available once hydrocarbon production ceases.
CCS is an important example of system integration because the existing gas infrastructure can be (partially) used for the transport and storage of CO₂. Furthermore, electricity is highly beneficial on platforms to compress, condition, and monitor CO₂, even though offshore CO₂ transport and storage power requirements are low compared to the power required for natural gas production. Thus, synergy can be achieved by electrifying oil and gas platforms and using them for CCS.
CCS is essential in the production of blue hydrogen, which is produced by reforming natural gas or residual gases from industry to hydrogen and CO₂. CCS could also contribute to the net removal of CO₂ from the atmosphere, for example, by applying CCS in combination with bioenergy (BECCS) or direct capture (DAC) of CO₂ from the air.
Example of CCS projects in the Netherlands
The Porthos project was set to start in 2024/2025 and will then store ~2.5 Mt/a of CO2 from industrial sources in the port of Rotterdam. The CO₂ will be transported to the offshore P18 depleted gas reservoir 20 km off the coast after being compressed on land.
Blue hydrogen
Blue hydrogen is produced by reforming natural or industrial residual gas while capturing the CO₂ emissions from the process. This CO₂ is then available for use or storage. This results in low direct CO₂ emissions. Blue hydrogen differs from grey hydrogen as the CO₂ released in the former process is captured and stored. It is an important hydrogen production route as it allows for fast and stable decarbonization. The considerable hydrogen production volumes already possible in the short term justify the (partial) conversion of the gas infrastructure for hydrogen.
Blue hydrogenis relevant for offshore system integration as it requires existing gas infrastructure to produce the natural gas needed for the process and requires legacy gas infrastructure that can be (partially) used for the transport and storage of CO₂. Also studies have been performed to develop offshore blue hydrogen facilities.
Service and logistics
It is important to note that in order to realize and operate the integrated energy systems, there are logistic and services requirements. As of now, the vessels and logistic services required for offshore wind and oil and gas activities are handled completely separately. Currently, significant attention is given to the costs, efficiency, and environmental footprint of offshore logistics services, which leads to a strong drive towards cost and emission reductions by optimizing installation, operation and maintenance (O&M) and reuse and decommissioning in the offshore wind and gas sectors. Sharing logistics services between offshore wind and the oil and gas sector is anticipated to result in emission savings and cost reductions. It may also reduce the pressure on scarce human capital in the future, supported by future autonomous inspection and O&M of offshore assets.